Advancements in Horticulture: Improving Crop Yield and Quality
Horticulture is undergoing a profound transformation, propelled by technological innovations that are enhancing crop yield and quality. These advancements are critical in addressing the global challenges of food security and climate change.
The integration of molecular techniques, precision agriculture, sustainable practices, and biotechnology is revolutionizing the way we approach plant breeding and farming. This article explores the cutting-edge methods that are setting new benchmarks in horticulture and paving the way for a more productive and sustainable future.
Table of Contents
Key Takeaways
- Molecular breeding and genomic selection have significantly shortened breeding cycles, improving crop yield, disease resistance, and stress tolerance.
- Precision agriculture, powered by digital technologies and AI, is transforming farming with real-time data and predictive analytics for better decision-making.
- Sustainable agricultural practices are being developed to reduce chemical inputs and enhance environmental resilience through innovative agronomic strategies.
- Advancements in breeding and biotechnology are creating high-yielding, climate-resilient cultivars, crucial for mitigating the effects of abiotic stress on crops.
- The future of horticulture lies in the integration of omics, marker-assisted selection, and biotechnology to promote sustainable and precision agriculture.
Revolutionizing Plant Breeding with Molecular Techniques
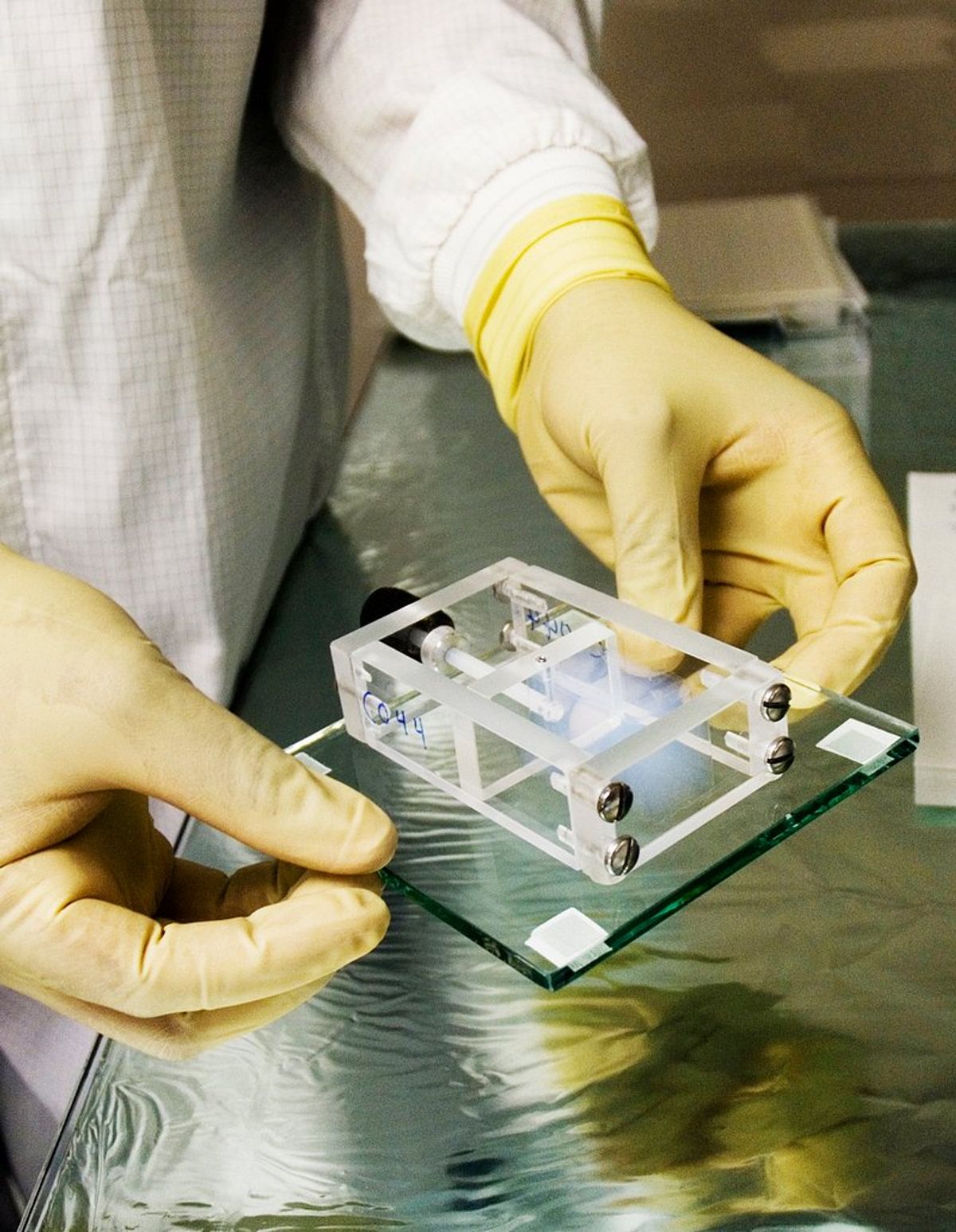
Accelerating Breeding Cycles with Genomic Selection
Genomic selection (GS) represents a paradigm shift in plant breeding, harnessing the power of the entire genome to expedite the breeding process. Unlike traditional methods that focus on specific genetic loci, GS utilizes a plethora of genetic markers, such as Single-Nucleotide Polymorphisms (SNPs), to predict complex traits like yield and stress tolerance.
The integration of GS into breeding programs has shown promising results in various crops. For instance, in sugarcane, GS has the potential to significantly increase the rate of genetic gain, mainly by reducing the breeding cycle duration. This is particularly beneficial for crops with long breeding cycles, where time is a critical factor.
The application of GS is not limited to accelerating breeding cycles; it also enhances the selection accuracy for desirable traits, leading to the development of superior cultivars.
The table below summarizes the impact of GS on different selection techniques:
Selection Technique | Impact of GS Integration |
---|---|
Single Plant Selection | Improved efficiency |
Single Seed Descent | Shortened cycles |
Marker-Assisted Selection | Enhanced disease resistance |
Clonal Selection | Accelerated cultivar release |
By embracing GS, breeders are not only able to reduce the time required to develop new varieties but also improve the overall quality and adaptability of crops to various environmental stresses.
Enhancing Disease Resistance and Stress Tolerance
The relentless challenge of shifting precipitation patterns and the rise in pest and disease outbreaks have made it imperative to develop crops with enhanced disease resistance and stress tolerance. Molecular breeding techniques are at the forefront of this endeavor, utilizing tools such as marker-assisted selection, genomic selection, and the revolutionary CRISPR-Cas9 genome-editing technique.
By identifying and selecting genes that confer resistance to both biotic and abiotic stresses, breeders are able to create varieties that are better equipped to thrive in changing environmental conditions.
Recent advancements have shown promising results. For instance, the overexpression of genes like LcFIN1 and MTP1 has led to increased tolerance in plants under abiotic stresses such as cold and heavy metal toxicity. The table below summarizes some of the key genes and the traits they have improved:
Transgenic Crop | Abiotic Stress | Gene and Its Source | Traits Improved |
---|---|---|---|
Tobacco | Salinity | bet A from Escherichia coli | Enhanced biomass |
Arabidopsis and tobacco | Multiple (Salinity, drought, cold) | GmbZIP1 from soybean | Enhanced tolerance |
Rice | Heavy metal | MTH1745 from thermophilic archaea | Improved photosynthesis and mercury tolerance |
These examples underscore the potential of genetic engineering in creating crops that can withstand various environmental challenges, thereby securing food production for future generations.
Integrating Bioinformatics for Advanced Crop Traits
The fusion of genotyping tools and advanced bioinformatics is revolutionizing the way we approach crop breeding. By analyzing genetic markers and identifying desirable traits, breeders can now develop crops with enhanced yields and resilience to environmental stressors. This precision in breeding is not only faster but also more accurate, ensuring that the resulting plants meet the specific needs of diverse ecosystems.
The capability to manage and interpret multi-omics data has led to significant advancements in plant phenotyping. As a result, we’re witnessing a new era of predictive breeding, where AI models contribute to the development of tailored forage varieties. This synergy between technology and agriculture is pivotal for global food security.
The integration of high-throughput phenotyping with bioinformatics has streamlined the management of large-scale genomic data, enabling more precise selection and breeding decisions.
Looking forward, the potential of omics-driven exploration is immense. It promises to yield more high-yielding tolerance genotypes, advancing sustainable and precision agriculture. The table below summarizes the impact of bioinformatics integration in crop breeding:
Aspect | Impact |
---|---|
Crop Yield | Increased |
Disease Resistance | Improved |
Environmental Tolerance | Enhanced |
Breeding Accuracy | More Precise |
Breeding Speed | Accelerated |
Precision Agriculture: The Digital Transformation of Farming
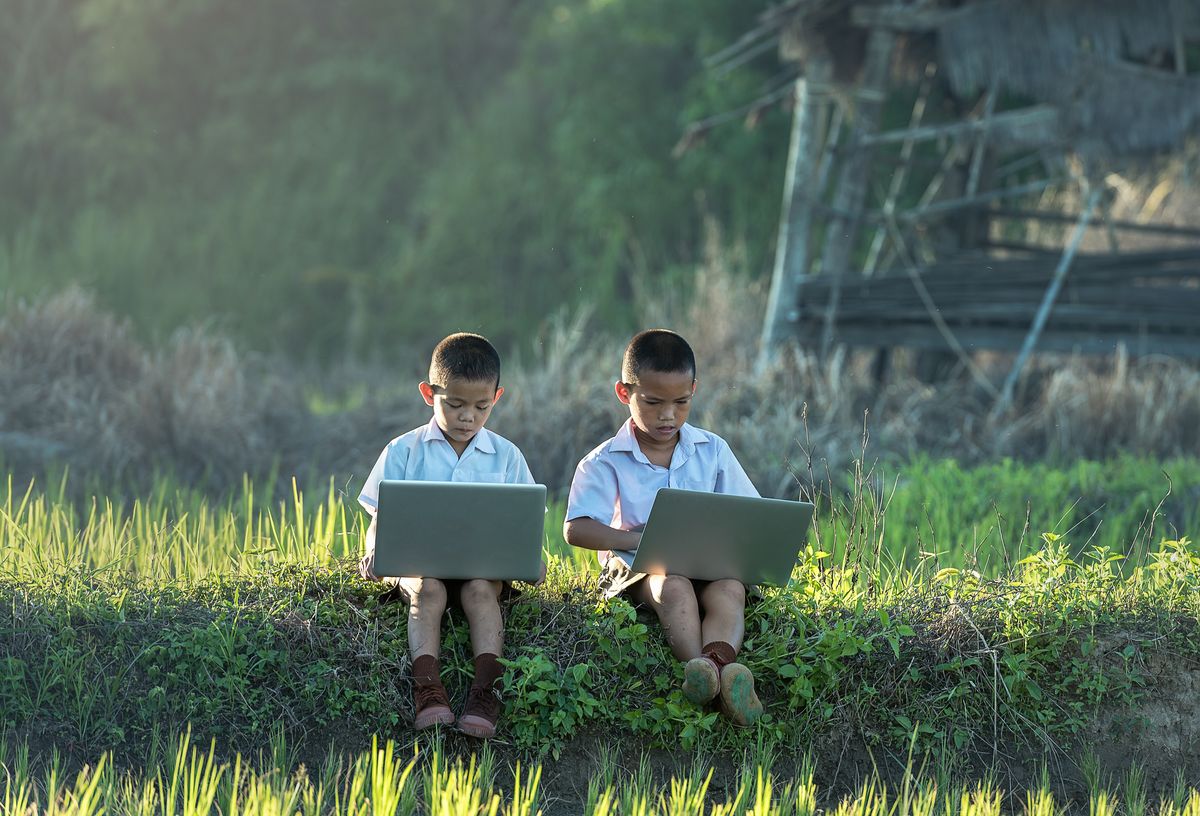
Adoption of High-Throughput Phenotyping
The advent of high-throughput phenotyping (HTP) has marked a significant milestone in the field of precision agriculture. By leveraging advanced technologies such as unmanned aerial vehicles (UAVs) and sophisticated machine learning algorithms, HTP enables the rapid and precise measurement of complex traits, like biomass yield, which are critical for enhancing crop performance.
Phenomics, the study of phenotypes on a large scale, has become integral to modern plant breeding strategies. The integration of phenomics with genomic information allows for a comprehensive characterization of resilience traits, streamlining the breeding process for targeted programs. This synergy between phenotypic and genomic data is a cornerstone in the development of crops that can withstand diverse environmental conditions.
The combination of HTP with bioinformatics tools has revolutionized the management of genomic data, leading to more informed and precise breeding decisions.
The table below summarizes the impact of HTP on plant breeding:
Aspect | Impact of HTP |
---|---|
Data Collection | Enables rapid, precise measurement of traits |
Breeding Programs | Facilitates targeted selection and breeding |
Crop Resilience | Enhances characterization of resilience traits |
As we look to the future, the potential of HTP, substantiated by AI technology, is vast. It promises to further refine predictive breeding and contribute significantly to the advancement of precision agriculture.
Leveraging AI for Predictive Breeding
The integration of Artificial Intelligence (AI) in predictive breeding is transforming the landscape of horticulture. AI serves as a powerful tool for the prediction of crop performance, enabling breeders to make informed decisions. By analyzing complex multi-omics data, AI facilitates the development of accurate predictive models that consider genotypic, phenotypic, and environmental data.
The concept of integrated genomic-enviromic prediction (iGEP) is a testament to AI’s potential in predictive breeding, encompassing a holistic view of plant performance.
The use of AI extends beyond data analysis to include the management of large-scale genomic data, streamlining the breeding process. This approach not only enhances the yield and resilience of crops but also addresses global challenges such as climate change and food security. As we move forward, it is crucial to establish ethical and regulatory frameworks to ensure the responsible application of AI in horticulture.
Real-Time Data for Informed Decision Making
The integration of real-time data into agricultural practices is transforming the landscape of farming. Predictive analytics, powered by machine learning, is enabling farmers to make more informed decisions that lead to optimized crop yields and resource management. For instance, price prediction models assist in determining the most profitable crops to plant, considering factors such as historical pricing and climate data.
- Real-time monitoring of crop health
- Predictive analytics for yield optimization
- Precision irrigation and fertilization
- Livestock health and productivity tracking
- Supply chain efficiency
These applications of real-time data are not only enhancing the precision of agricultural operations but also contributing to a significant reduction in waste. As the digital agriculture market is projected to reach a value of $4.0 billion by 2026, the adoption of data-driven solutions is set to become even more prevalent.
The use of aerial imagery and weather sensors feeds valuable data into algorithms, which then inform decisions on crop management and disease prevention. This proactive approach is crucial for maintaining high standards of crop quality and yield.
Sustainable Practices to Mitigate Environmental Stress
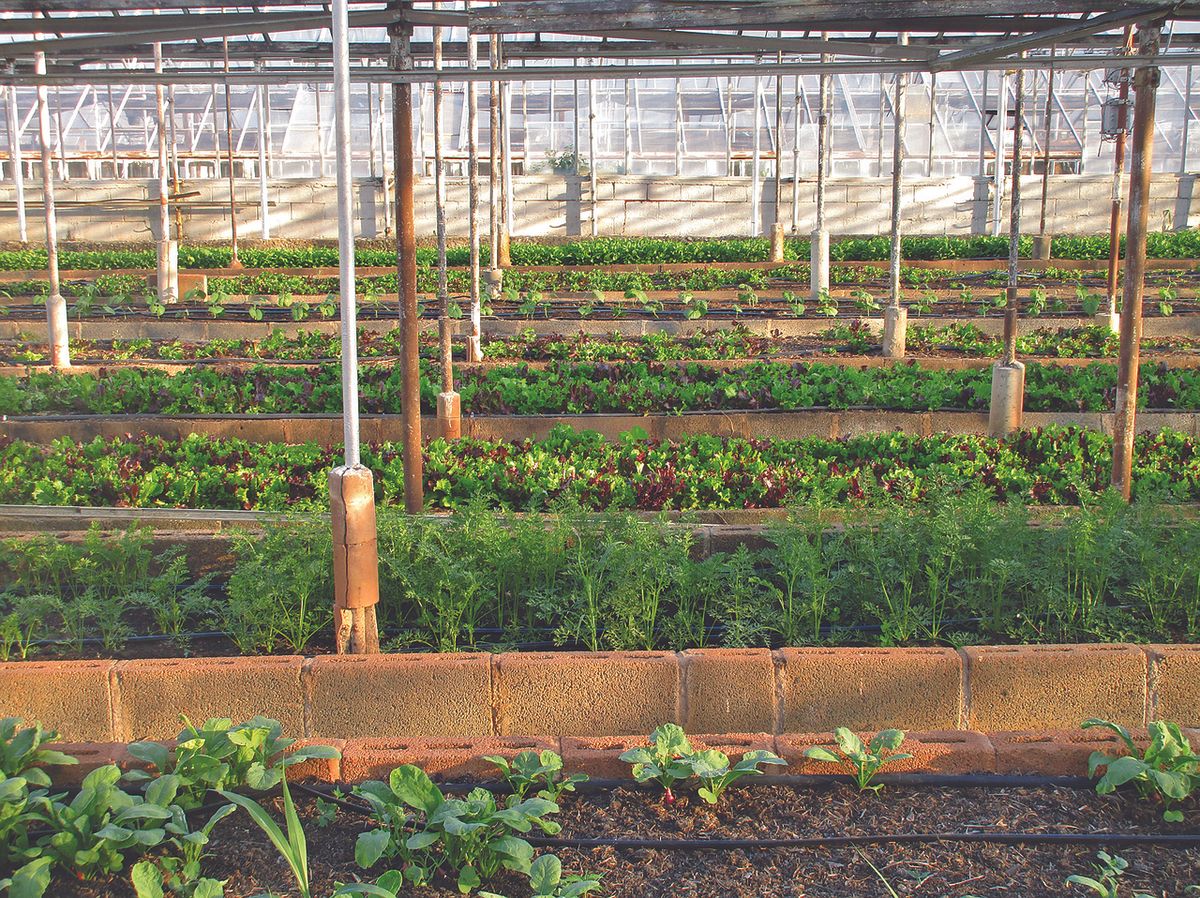
Developing Climate-Resilient Cultivars
The escalating challenges posed by climate change, such as extreme weather events, shifting precipitation patterns, and increased pest and disease pressures, underscore the urgency of developing climate-resilient cultivars. These cultivars are designed to withstand the unpredictable conditions and ensure sustainable crop production.
Breeding strategies are pivotal in creating varieties that can thrive under the duress of climate change. Both conventional breeding techniques (CBTs) and new breeding techniques (NBTs) are instrumental in this endeavor, offering a spectrum of tools to enhance crop resilience, particularly within low-input production systems.
The integration of molecular breeding techniques, like marker-assisted selection, genomic selection, and CRISPR-Cas9, has revolutionized the process. These methods enable the precise identification and selection of genes that confer resistance to biotic and abiotic stresses, paving the way for the cultivation of robust forage crops.
Screening germplasm under natural conditions is essential to identify tolerance accessions that can be utilized in breeding programs. To safeguard these valuable genetic resources from genetic erosion and the effects of climate change, proper conservation through in situ and ex situ methods is imperative.
Innovative Agronomic and Microbial Strategies
In the quest for sustainable agriculture, innovative agronomic and microbial strategies are pivotal. Microbial seed treatment and microorganism inoculation are at the forefront, enhancing abiotic stress tolerance in crops. These methods, along with grafting and the use of osmoprotectants, form a suite of techniques that improve plant resilience.
Emerging strategies such as the application of biochar, yeast extract, and seaweed extract, as well as agri-nanotechnology, represent a new wave of cost-effective and environmentally friendly options.
Collaboration among researchers is crucial to unlock the full potential of these technologies. The integration of biofertilizers, vermicomposting, and green manure, coupled with precision agriculture, offers a holistic approach to soil nutrition and pest management. Mixed cropping and crop rotations further contribute to this sustainable model.
The table below summarizes key innovative strategies and their contributions to enhancing plant tolerance under abiotic stress:
Strategy | Contribution |
---|---|
Microbial Inoculation | Enhances root development and nutrient uptake |
Grafting | Improves water and nutrient efficiency |
Biochar | Increases soil fertility and water retention |
Seaweed Extract | Boosts plant growth and stress resistance |
As we continue to face the challenges of a growing global population and the need for increased food security, these innovative practices are essential in our arsenal against abiotic stress, ensuring the sustainability and productivity of our agricultural systems.
Reducing Chemical Inputs through ICT Solutions
The reliance on chemical fertilizers for enhancing crop yields is a double-edged sword, with potential environmental and health repercussions. Information and Communication Technology (ICT) offers a sustainable alternative, enabling farmers to optimize the use of inputs and reduce reliance on chemicals.
ICT solutions provide farmers with access to critical data on weather patterns, soil moisture levels, and plant health, which are instrumental in making informed decisions about crop management.
By leveraging ICT, farmers can improve the efficiency of fertilizer use, as evidenced by studies in Vietnam and China. The integration of ICT in agriculture has led to the adoption of Precision Agriculture, which tailors farming practices to the specific needs of each plot, thereby minimizing waste and enhancing productivity.
Here is a summary of the benefits of ICT in agriculture:
- Enhanced decision-making through real-time data
- Reduced environmental impact by minimizing chemical usage
- Increased efficiency in fertilizer application
- Better market integration for small-scale farmers
- Improved crop yield and quality through precise agricultural practices
Enhancing Crop Performance Under Abiotic Stress
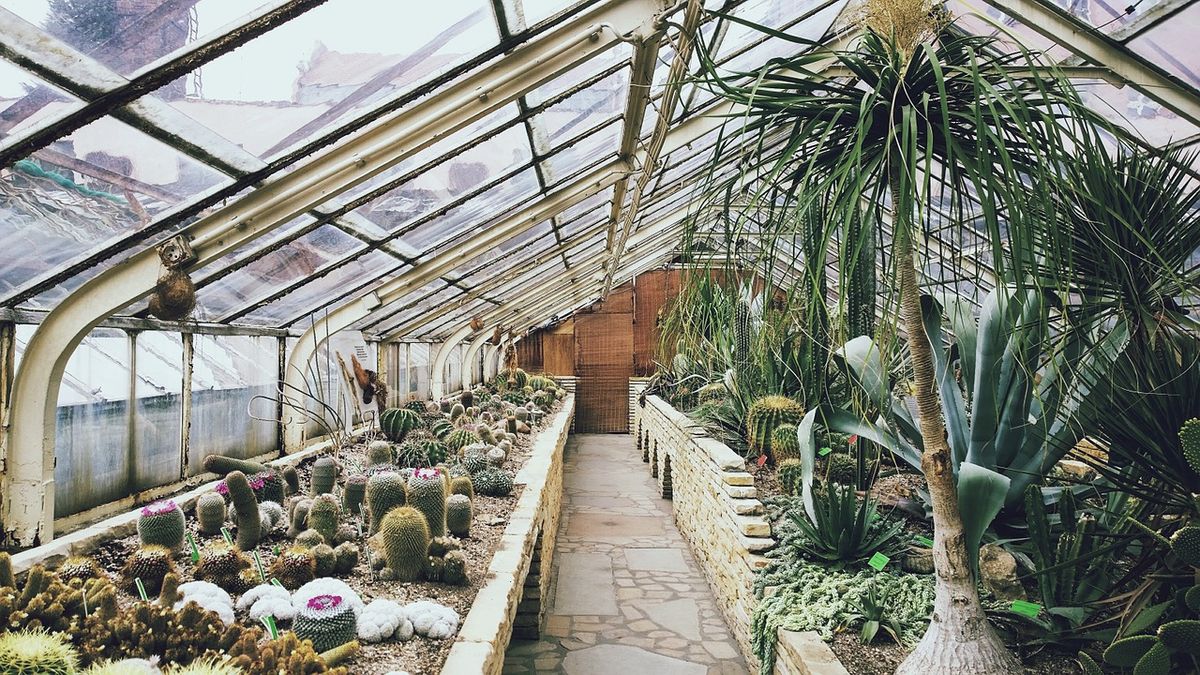
Breeding Techniques for Improved Tolerance
The quest for enhanced crop tolerance to abiotic stresses has led to the fusion of conventional and modern breeding techniques. Speed breeding is emerging as a transformative approach, significantly reducing the time required to develop tolerant cultivars. This method, along with the use of plant growth regulators and osmoprotectants, represents a leap forward in horticultural science.
The integration of various breeding methods, including pedigree selection, recurrent selection, and hybridization, is pivotal in generating resilient lines and cultivars. These techniques are instrumental in screening and identifying cultivars that can withstand harsh environmental conditions.
The table below summarizes the conventional breeding methods and their impact on crop tolerance:
Method | Description | Impact on Tolerance |
---|---|---|
Pedigree Selection | Tracking the ancestry of plants to select desirable traits. | Enhances specific tolerance traits. |
Recurrent Selection | Repeatedly selecting and interbreeding individuals with desired characteristics. | Increases overall genetic tolerance. |
Hybridization | Crossing different strains to combine traits. | Creates cultivars with combined tolerance from both parent strains. |
While these methods have traditionally required extensive manpower and time, advancements in technology are now enabling more efficient and precise selection processes. The goal is to not only improve tolerance but also to contribute to sustainable and precision agriculture, ultimately increasing farmer income.
Seed Priming and Microorganism Inoculation
Seed priming is a pivotal pre-sowing strategy that prepares seeds for better germination and establishment. Seed nano-priming, particularly with agents like Zinc Oxide nanoparticles, has shown to enhance nutrient uptake and improve the plant’s antioxidant properties, which are crucial under abiotic stress conditions. This technique can lead to increased soluble sugar and starch concentration in germinated seeds, as well as boost the activities of enzymes involved in germination.
Seed priming techniques vary, including hydropriming, osmopriming, biopriming, and molecular priming. The choice of technique is influenced by the plant type and environmental factors. For instance, melatonin used as a priming agent can confer heat tolerance and induce heat shock proteins, while priming with nanoparticles enhances seedling germination and various morpho-physiological traits.
The effectiveness of seed priming is evident in its ability to initiate the germination process without radicle emergence, leading to higher germination rates and uniformity among seedlings.
Inoculation with beneficial microorganisms, such as endophytic Bacillus subtilis, can act as a biostimulant, influencing germination and seedling growth. This symbiotic relationship between plants and microbes is an innovative approach to bolster plant resilience against environmental challenges.
Utilization of Plant Growth Regulators
The strategic application of plant growth regulators (PGRs) is a critical component in enhancing crop performance under abiotic stress. PGRs often modulate plant defense and can protect the plant from environmental threats by regulating reactive oxygen species (ROS) activity, chlorophyll metabolism, and other physiological processes.
For instance, the use of osmoprotectants such as polyols, glycine betaine (GB), and salicylic acid (SA) has been shown to improve the resilience of crops by enhancing antioxidant enzymes and osmotic adjustment. Notably, under water-deficit conditions, abscisic acid, uniconazole, brassinolide, and jasmonic acid have been applied exogenously to increase plant productivity.
The utilization of PGRs and osmoprotectants is not just about stress mitigation; it’s about empowering plants to thrive in challenging environments.
The following table illustrates some of the PGRs and osmoprotectants that have been used to enhance plant abiotic stress tolerance:
Abiotic Stress | PGR/Osmoprotectant | Crop | Mechanism | References |
---|---|---|---|---|
Salinity | 5-Aminolevulinic acid | Cotton | Enhanced seedling germination | [26] |
Drought | Melatonin | Wheat | Enhanced seedling vigour and tolerance | [138] |
The Future of Horticulture: Integrating Omics and Biotechnology
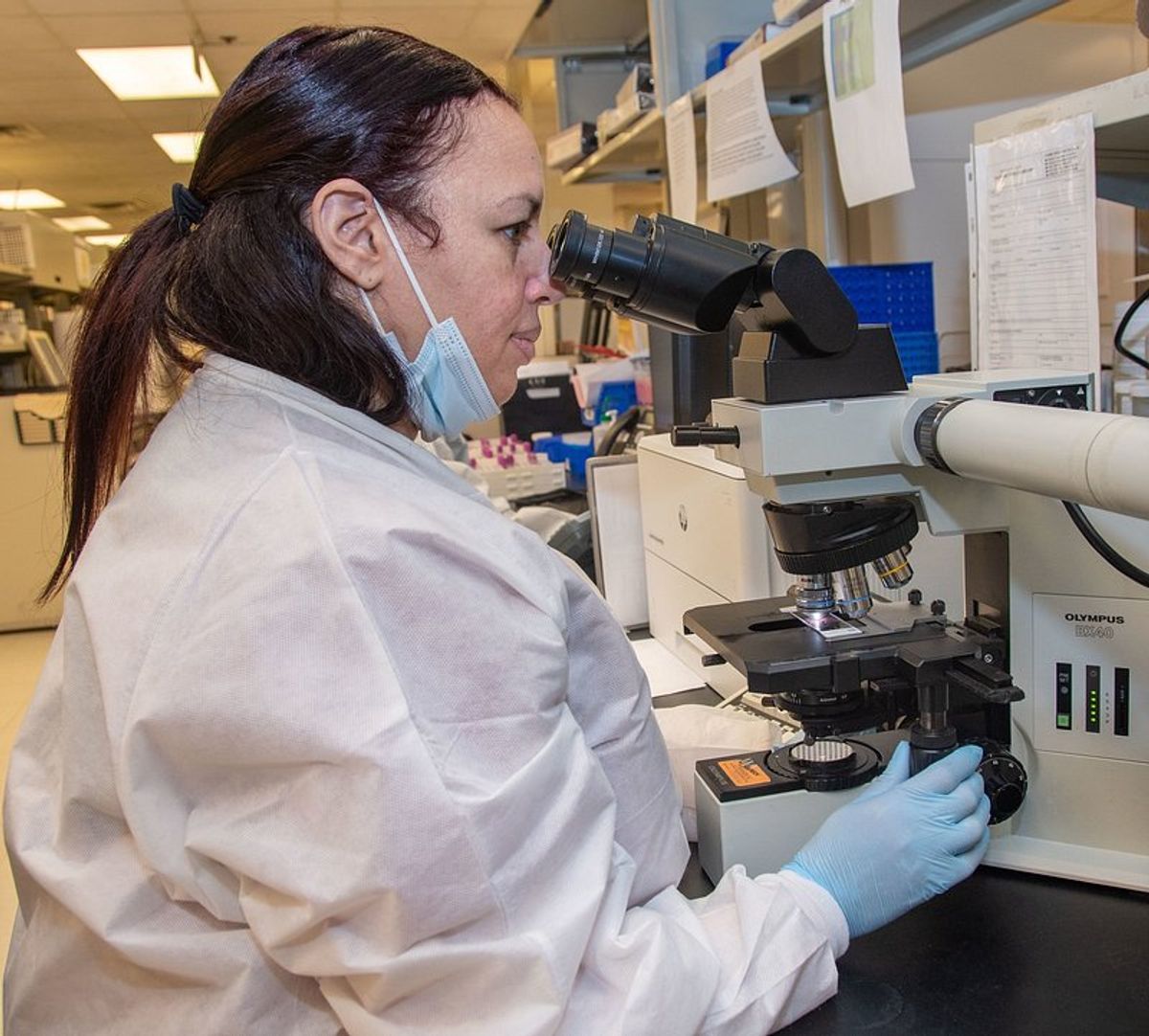
Omics for High-Yielding Tolerance Genotypes
Omics technologies are pivotal in the quest to develop genotypes with enhanced yield and tolerance to various stresses. By dissecting the complex interactions at the genomic, proteomic, and metabolomic levels, scientists are able to pinpoint the traits that contribute to robustness and productivity in crops.
The integration of omics with traditional breeding methods is revolutionizing the way we approach crop improvement. It allows for a more precise selection of traits, leading to the creation of varieties that are not only high-yielding but also resilient to environmental challenges.
The table below showcases examples of genes from model organisms that have been successfully introduced into crops to improve tolerance to specific abiotic stresses:
Crop | Stress | Gene | Outcome |
---|---|---|---|
Potato | Salinity | P5CS from Arabidopsis | Increased proline content and tuber production |
Tomato | Heat stress | StGLP from yeast | Improved tolerance to gradual heat stress |
Tall fescue | Salinity | AtNHX1 from Arabidopsis | Increased shoot and root dry weight |
Rice | Waterlogging, salinity, and drought | OsARD1 from rice | Increased ethylene synthesis and conferred tolerance |
In addition to genetic engineering, the application of nanomaterials, osmoprotectants, plant growth regulators, and nutrient management are also contributing to the enhancement of plant tolerance under abiotic stresses. This multifaceted approach ensures the development of more high-yielding tolerance genotypes, which are essential for sustainable and precision agriculture.
Marker-Assisted Selection (MAS) in Crop Improvement
Marker-Assisted Selection (MAS) has revolutionized the way plant scientists improve crop traits. By identifying markers closely linked to desired traits, MAS offers a quick and affordable path for genetic enhancement. This technique is particularly effective in introducing tolerance to various abiotic stresses, such as drought and salinity, which are critical for the resilience of crops like rice and wheat.
The process of MAS involves several steps:
- Identification of DNA markers associated with beneficial traits.
- Integration of these markers into breeding programs.
- Selection of offspring carrying the desired traits through marker screening.
- Repetition of the cycle to enrich the cultivar with favorable alleles.
The simplicity and efficiency of MAS make it an eco-friendly option that saves time and resources while ensuring the development of durable cultivars.
Recent advancements have seen the integration of MAS with speed breeding techniques, accelerating the breeding process and enhancing the development of cultivars with multiple stress resistances. The synergy of these methods is paving the way for the next generation of high-yielding, stress-tolerant crops.
Advancing Sustainable and Precision Agriculture
The integration of sustainable practices with precision agriculture is pivotal for the future of farming. Farmers are increasingly adopting digital technologies to optimize resource usage and reduce environmental impact. This synergy enhances efficiency and productivity across various agricultural domains, including irrigation, weeding, crop monitoring, and spraying.
Precision agriculture’s role in sustainable farming extends to the promotion of biofertilizers, green manure, and mixed cropping systems. These practices contribute to soil nutrition and pest management while conserving water and improving crop rotations.
Precision agriculture varies significantly across regions and crops, reflecting the diverse needs and capabilities of farmers worldwide.
The table below illustrates the adoption of precision agriculture in different regions:
Region | Adoption Level |
---|---|
North America | High |
Europe | High |
Developing Countries | Variable |
As we navigate the present and future of farming, it is clear that the adoption of precision agriculture is not uniform. In developed countries, particularly in North America and Europe, the uptake is significant. However, in developing regions, the level of adoption can vary greatly, influenced by factors such as economic resources and access to technology.